It would probably have been the science story of the year—any other year. In January 2020, a team of biologists, roboticists and computer scientists announced that they had created the world’s first living robots. These Xenobots were cells culled from a frog, sculpted with the help of an evolutionary algorithm, and then set free to roam under the microscope. Liberated from the constraints of frogness, these cells had designs of their own. They collaborated. They interacted. They performed basic tasks. And eventually, in more recent experiments, they started to multiply.
This is the team’s latest revelation: Xenobots can autonomously assemble identical copies of themselves from individual cells floating in the spring water around them. The bots tipped off their creators by pushing around bits of debris. “They started corralling inert particles. I saw them making heaps,” says Tufts biologist Michael Levin. “They’re basically making this Xenobot in front of our eyes.” His colleague, University of Vermont roboticist Josh Bongard, elaborates: “it suggested this competency may be latent to the material.”
Xenobots may one day become useful for performing microscopic tasks, like transporting medicine within the human body, or studying the root system of a plant. But the team around Bongard, Levin, and their colleagues, Tufts biologist Doug Blackiston, and Wyss Institute computer scientist Sam Kriegman, are interested in much more than the utility of these new beings. In their eyes, Xenobots open a whole new set of questions of how we think about cognition, cells, and the smallest iterations of the evolutionary drive.
What do Xenobots reveal about the evolutionary charge in every cell? What can they teach us about the origins of cognition, and how we could replicate it? More practically, how has the Xenobot team’s thinking about the implications and applications of their discovery evolved over the past two years? I sat down virtually with Levin and Bongard to find out.
Max: What is your overarching goal in creating and studying these living robots?
Michael: We are broadly interested in where and how minds form in the world. I mean that both on an evolutionary scale, in terms of basal cognition—what are the minimal creatures that exhibit some form of agency, memory, intelligence?—and on an ontogenic scale. We all start life as a single cell, right? Molecular biologists like to see each cell as a chemical machine. These cells stack up en masse until we arrive at something like a conscious state. How does that journey take place? What does it mean for things to have minds? Where can they come from? And how do we engineer them?
Max: As a roboticist, Josh, do you find yourself interpreting these fundamental questions differently than a biologist?
Josh: Mike and I share a lot of intellectual interests. And one of them is this synthetic approach. If we want to understand minds in whatever substrate—if we really know what we’re talking about—we should be able to create it. It’s sort of science through synthesis, rather than analysis.
Max: What’s an example of something that you’d want to know by “creating it”?
Josh: Pick ten of our colleagues from biology, AI or robotics, and ask them to define what a mind is and you’re going to get ten different definitions. And each of those folks, myself included, will be confident that our definition is correct. The whole history of AI since the Second World War is people saying we know how to recreate minds—it’s going to take us a couple years, no problem. 74 years later, we’re still struggling. It feels like we know what we’re talking about, but when you get down to brass tacks, things get really difficult. For me, that’s why you have to build it. That’s the only way to end these arguments once and for all.
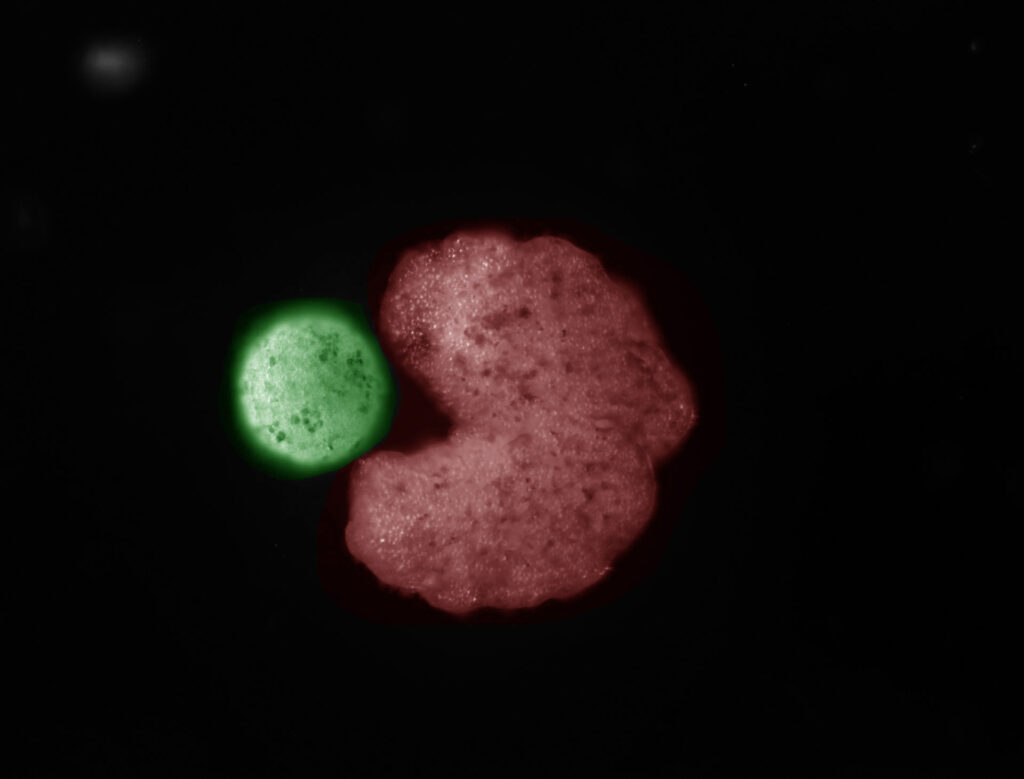
Max: Your team demonstrated last year that Pacman-shaped Xenobots “parents” can collect individual cells floating around them and assemble them into copies of themselves. In the paper, you use the term “replication.” Others have used the term “reproduce.” Why did you settle on this more asexual terminology?
Josh: We tried to avoid “reproduction” in the paper—”replication” is a little less controversial. To a roboticist, the term is perfectly apt. Back in the ‘40s, John von Neumann said that if machines ever make copies of themselves, they’re probably not going to grow in volume and mass and then split into two, or grow a little machine inside themselves and then release it. They’ll reach out in the environment, find raw materials, and combine those materials externally through motion. If you choose to view the Xenobot as a robot, this makes perfect sense from a technical point of view.
Michael: There are two ways you can go. You can try to shoehorn your phenomenon into existing terms and try to obey what you think people understand by this. Or you can bite the bullet and say the terms have to fit the reality, not the other way around. Xenobots are not an actual biological lineage, because they don’t have strong heredity. But they definitely do make copies of themselves. So, we need a word for that. Do we use an existing word and try to pull everybody along in a particular direction, or do we coin a new word? In this case, we didn’t.
Max: What’s wrong with coining new words?
Michael: Somebody once said to me, ‘You know, you could avoid all the fighting with everybody about cell memory if you just came up with a different word. Call it shmemory and then say that cells have shmemory, and then nobody has to fight about it.’
Sometimes that’s the right move. But the problem with that is you miss out on the unification. What I told her at the time was “Okay, and Isaac Newton should have said, The moon—that’s gravity—and this apple here that’s shmavity.” What did you just miss out on? The whole point of it, right? This is a hill I’m willing to die on: Cells have actual memory. They don’t have some shmemory, they have actual memory. In this case, we didn’t want a different word. It really is replication.
If this isn’t intelligence, I don’t know what is.
Michael Levin
Max: Xenobot replication involves one agent assembling pieces into a copy, which is quite different from sexual reproduction. Is sexual reproduction possible in Xenobots?
Michael: I think it would take a lot to make that happen. Not that it’s impossible. A more interesting aspect of this is that the genome in the cells has been honed to create a machine that reproduces in a particular froggy manner, right? It’s got all the stuff you need to reproduce sexually. We then mess with it and basically make it impossible for it to do any of that—we take away everything that’s not a skin cell. Within 48 hours, this thing solves the problem. It figures out a new way to replicate even though it’s not quite as fancy as the original. It solved the problem in an entirely new way, which, as far as we know, no other animal on earth does. There’s never been evolutionary pressure to be able to do this. To me, that’s just mind-boggling. If that’s not intelligence, I don’t know what is.
Max: Can you let these things replicate in perpetuity?
Michael: So “we don’t know” is one answer. It’s kind of funny, we didn’t spend much time optimizing the conditions at all. We just sort of let them go under natural conditions. They’re swimming around in spring water. There are no nutrients in that water. What powers them are these sort of yolk platelets that they get from the mother. When they use up that energy store, they’re done. It’s entirely possible that they’re simply running out of energy, and that if we were to feed them, which we do know how to do, maybe they would have the energy to make bigger piles in perpetuity.
Josh: We tried around a little bit with the concentration of dissociated cells. We tried to give them more components—there were lots of cells strewn around. That helped a bit but there were diminishing returns.
Max: You’ve shown that “parent” Xenobots can assemble many identical cells into a large clump that resembles themselves. Could these Xenobots assemble multiple different components into a more complex creature than what you’ve shown?
Michael: Yeah, absolutely. At every step of the game, you could imagine introducing different cell types.
Josh: It’s interesting to think about adding different cell types or all the other things we can throw in. But it’s also really important to remember that the frog skin cells themselves are very competent. They seem to be able to do things that we didn’t think they could do before. As a roboticist, if you’re working with dumb materials—non-living materials—you pretty quickly exhaust what, say, metal and ceramics can do. You’ve got to go looking for other materials. You’ve got to find more clever ways to put them together.
Max: But in this case, you’re not working with dumb materials. How does that change your process of building a robot?
Josh: One of the interesting things for me in this project is to resist that impulse of putting more stuff into the stew. Put the AI and frog cells together—how far can we get with just that? And the answer seems to be: pretty far. I think that’s a really interesting way to get at intelligence from a different angle. They will reorganize, they’ll figure out how to do things in novel combinations—let’s see what they can do before we put in nanoparticles and genetic modification.
Max: The Xenobots that replicate in this work are millimeter-scale. How big could Xenobots get?
Michael: There’s a couple of different size limits. One size limit is how large it could get before it’s too heavy for the cilia to move it around. Another thing is a very kind of familiar size limit in bioengineering, which is about one millimeter. That’s how big you get before the inside starts dying because it’s not getting oxygen.
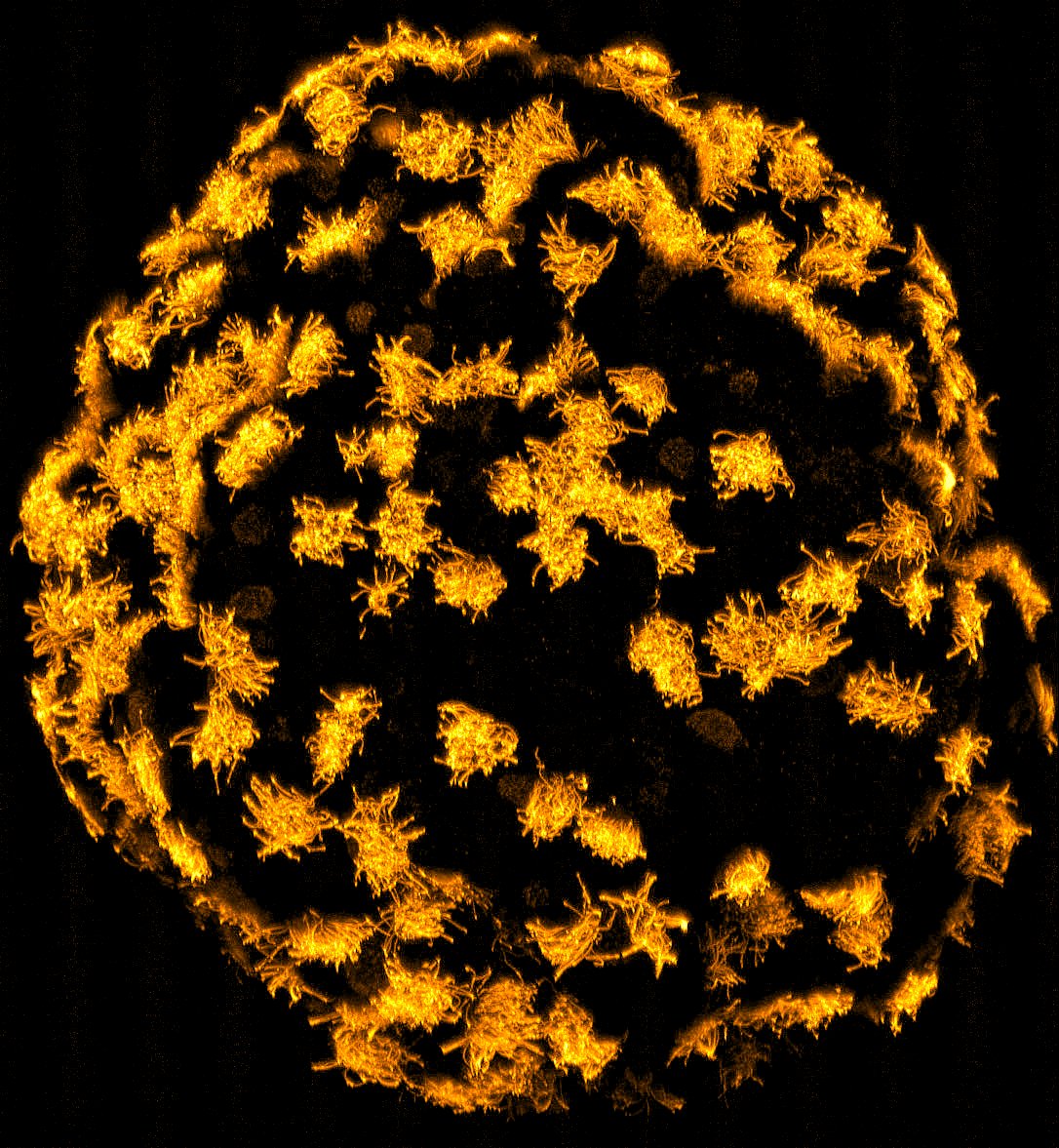
Max: How do you get around that?
Michael: You could imagine making something that’s like a Sierpiński gasket, that has holes all through it, kind of a fractal with big and little holes. If a medium can go through that, I think that would probably be huge news. If you give it a vasculature, it could obviously grow much, much bigger.
Max: Could a scaffold like that be made of cells, or would it be non-biological?
Michael: I think you could do it biologically. I mean, look at sponges. There are organisms that have that architecture already. I’m not saying we know how to make that kind of scaffold today, but I see no reason why we couldn’t make it eventually. For the early work, you know, I specifically wanted to avoid nanomaterials and genomic editing. You put all that stuff in, and then people say: You’ve engineered some stuff, fine. But what gets lost in all of that is how much of the heavy lifting the cells are actually doing themselves.
Max: How important is it to push the limit on size then?
Josh: From a robotics point of view, I’d say it’s actually not that important. The fact that we can make millimeter-sized robots in a couple of days—that’s transformative for the robotics industry. We’ve been trying to design robots for the longest time that can access hard-to-reach places. You want to inspect the root system of plants in a vertical farm or hydroponic plant. You want to tunnel through cultured meat as it’s being grown. You want to inspect underwater vents, filters. You name it.
We can now start exploring how to create intelligent machines.
Josh Bongard
Max: Where specifically could millimeter-scale robots be used?
Josh: Inspection tasks, basically. The canonical example is the Roomba. The Roomba is the most commercially successful robot because it gets somewhere that’s hard to reach, which is under couches.
Michael: One of the other applications for all this has nothing to do with the bots themselves. And that’s cracking the morphogenetic code, really understanding how it is that collectives of cells make decisions about what they’re going to do. Practically speaking, they could contribute greatly to regenerative medicine.
Max: What role could autonomous Xenobots play in regenerative medicine?
Michael: At some point, we’re going to want to make a hand for somebody. And that hand has to be this size. So, we are going to have to understand what is involved in dictating and enabling things to grow to a particular size.
Max: And that means controlling differentiation of stem cell bots?
Michael: It’s not published yet, so I can’t really talk about it, but I will just say basically there’s nothing about this that depends on them being stem cells.
Max: How, then, do you conceive of complex assembly?
Michael: The most obvious one is basically to recapitulate early embryonic development. At one point, you had some cells, and those cells together made a decision that they were going to be a hand—not a fin, not a heart, and not a tumor. Everything took off from there.
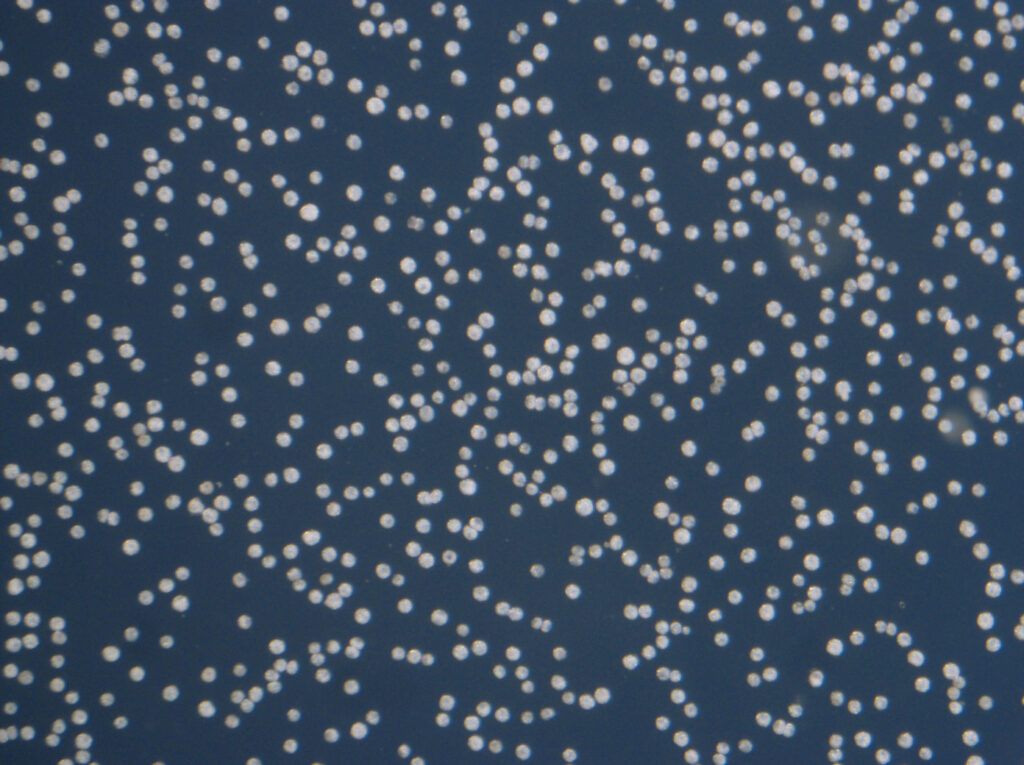
Max: Your team recently published evidence that you can regenerate frog legs, which don’t normally regenerate, by intervening in the first 24 hours, then doing nothing for a year and a half. Why was that the best approach?
Michael: Because you’re not trying to micromanage the process. You’re trying to guide the decision within the first 24 hours. They already know how to build a leg. So that’s one of the goals here. We want to understand the sub-routines. What are the modules that we can trigger? Can we say build a hand, build an eye? Then maybe somebody wants flippers or tentacles instead of a hand. What would it take to make that?
Max: What can a roboticist learn from embryology?
Josh: Many, many, many things. This is form and function that changes over time. 99.999% of machines, we make—robots, cars, infrastructure— you build it, and it never changes. Our whole approach to engineering, and our technological civilization, is to make machines that do not change. A lot of my robotics colleagues make a 300-pound, six-foot humanoid form, and then they pour AI into it, cross their fingers, and hope for the best. Not to cast aspersions on my colleagues, they’ve made some amazing progress. But that’s about as far estranged as possible from how biological humans develop. We’ve been limited. You can’t grow steel; you can’t grow and duplicate plastic. We can now start exploring how to create intelligent machines—where we give them a hint at the beginning and that projects the way that they grow and how they respond to their environment, so that they set into a particular form and function that’s useful to us.
Max: What have you learned from these Xenobots about life and intelligence? What are your main takeaways.
Josh: Life always finds another way to do what it needs to do. Everyone has their own definition of intelligence. I don’t like any of the definitions. The one I hate the least is “make sure you don’t get painted into a corner in the future.” One of the things I’ve learned in working with Mike’s group and the Xenobots is the staggering breadth of how life is capable of that—how good life is at making sure that it doesn’t get painted into a corner in the future.
Michael: What I’m really looking at is the idea of intelligence throughout evolution. The idea that evolution does not apparently create solutions to specific environments, it creates machines that are competent at solving problems in novel circumstances. This, to me, is the root of intelligence. Biology has it all the way, from the bottom up. The evolutionary process itself can be said to have a degree of intelligence. And then all the things that it produces have intelligence as well. Fleshing that out is, I think, the greatest challenge going forward.
The conversation has been lightly edited for clarity.